Neuroscience
Perceptual Learning Stabilises Action: A Test of the Bingham Model
Bingham's perception-action model was initially inspired by perceptual judgement studies (using vision and proprioception). The HKB phenomena are movement phenomena, however; simply noting that the same qualitative pattern is seen in different judgement and action studies is a good first step but only suggestive, at best. We therefore next took simultaneous judgement & action measures from a movement task where we manipulated the feedback display (Wilson et al, 2005a). For instance, when the display showed 0°, movement was stable, even when the movement was at, for example, 90°. Perception of relative phase was driving the stability of the movements.
On the basis of all this data, the model predicts that the reason 0° and 180° are easy is that the information specifying that you are moving this way is easily perceived. There is provisional evidence to support relative direction of motion as the specifying information (Bogaerts et al, 2003; Wilson et al, 2005b; Wimmers et al, 1992) with relative speed acting as a noise term. This variable certainly predicts the observed pattern, as the relative direction of motion is only stable at 0° and 180°. It is maximally variable at 90°, which would explain why movements here are also maximally unstable. The model is therefore explaining the problem with moving at 90° as a problem detecting the information required to maintain the coordination; as we saw in the case of friction, no information means unstable behaviour.
The model therefore makes a critical prediction. If we could improve people's ability to perceive 90°, they should gain the ability to move at 90° without any practice at the movement itself. All previous learning studies had entailed training people to move by having them move, with the help of various forms of transformed feedback methods (visual metronomes or Lissajous plots; more on this when I discuss feedback). The prediction, that movement stability should improve with improved perceptual ability, is a strong test of both the model and the modelling strategy in general, and the experiment to test it was the first half of my dissertation.
The experiment itself was actually very straight-forward, although time consuming to run.
Baseline
We took Baseline measures of action stability by having participants use a joystick to control a dot on the screen so as to move at 0°, 180° or 90° to an oscillating dot on the screen (Figure 1). We measured movement stability as the proportion of time spent at the target relative phase, +/- 20°. Everyone produced the standard HKB pattern (good movement stability at 0°, good but lower stability at 180°, terrible at 90°; see the black lines in Figure 3). We split the participants into two groups of 6 (Learning and Control), balanced according to their ability to move at 90°.
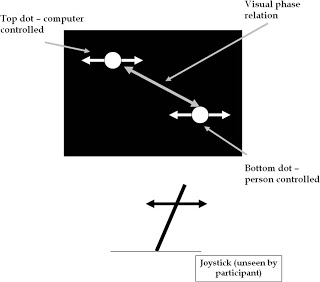 |
Figure 1. Schematic of the setup for the action task |
We also took Baseline measures of perceptual ability at 90° and 180°. Participants viewed two displays, one moving at the target relative phase and the other at some different relative phase; the magnitude of the difference on each trial was randomly selected from 10 alternatives. This is a two-alternative forced choice (2AFC) psychophysical procedure, using a non-adaptive staircase. We computed the difference required before people could reliably tell the difference between the two displays (75% correct) and used this as an estimate of the threshold. See Figure 2.
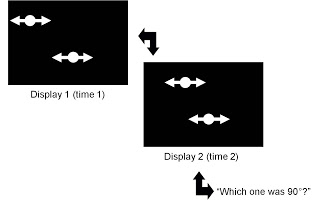 |
Figure 2. Schematic of the judgement task. |
Thresholds at 180° were approximately 11°, i.e. the displays needed to be different by 11° before participants could reliably tell the difference. Baseline thresholds for 90° were 24° - much higher, and also highly variable both within and between participant. This matches nicely to the original visual perception study results.
Training
We then extensively trained the 6 people in the Learning group on the perceptual judgement task until their thresholds settled out and stopped changing. This took between 7 and 14 sessions, so the training was time consuming. The training had the participants make increasingly difficult discriminations of 90°; they received 'correct'/'incorrect' feedback and were shown 90° again when they made an error. There was no exposure to the movement task.
Thresholds at 90° steadily decreased over training, and settled out at 12°, very similar to performance at 180°.
Post Training and Retention
These sessions repeated the Baseline; Post Training was at least 1 day after the final training session, and Retention was at least 1 week after.
The question was simple: had the Learning group improved in their ability to move at 90°? What about the Control group, who did the same number of movement trials - had they improved at 90° just by trying the movement?
The results were straightforward: only the Learning group improved their movement stability, and only at 90° (the learning did not generalise) - see Figure 3.
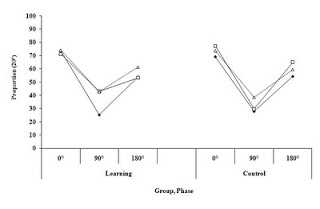 |
Figure 3. Movement stability data from the three Assessment sessions |
The conclusion was straight-forward: the Learning group was able to use their improved perceptual ability to maintain movement stability. As we noted in the paper, this is not a case of learning generalising from the perceptual to the action domain: there is only the perception-action domain, and the training altered the perception-action system. This experiment, therefore, clearly demonstrated that movement stability is a function of perceptual ability; 0° isn't stable because there's an attractor there (as per the dynamic pattern hypothesis), there's an attractor there because 0° is stably perceived!
Some comments
- There were two ways, in principle, that the perceptual learning might have helped perceive 90°. First, the training could have improved people's discrimination of the relative direction of motion. However, we noted that at 90° this is unavoidably variable and noisy, and so it might not be possible to ever improve detection of it. The alternative is to learn to use a new information variable, one which is detectable and specifies 90°. In support of this, perceptual learning at 90° did not transfer to perceptual or action performance at 0° or 180°. We directly tested this in the second half of my dissertation, which I will talk about next.
- The training was extensive and tedious, and the returns were, frankly, small. While we did demonstrate the three-way statistical interaction between relative phase, session and group to support our conclusions, the improved movement stability at 90° was small (especially in the context of the action training we have done since, and which I will also blog about soon). I have some hypotheses about why all this is, but I have yet to test them directly.
- The data reported here was actually a replication of my dissertation work. We reran the study to tidy some of the methodology and get the key three way interaction (which was not quite significant in the original 8 participants). The reason the interaction did not come out previously was that we actually found a slightly different pattern of results; while the Learning group did show significant improvement (at 90° only) over Baseline at Retention, there had been no improvement by Post-Training. At the time I attributed this to some form of consolidation, and suggested the perceptual training needed to be used in the context of an action before the overall change to the task dynamic occurred. However, we didn't find this pattern in the replication, and the reason was the training was much more extensive this time round - we pushed the learners much harder and longer before testing their movement. I'm convinced there's something quite interesting here, and will be pursuing this in the future.
- There is some other recent work on this topic now as well from Nicola Hodges, with mixed results (finding an effect on movement; Feijen et al, 2010; or not finding an effect on movement, Maslovat et al, 2010). I think there are reasons for the discrepancies, including insufficient training and specific methodological differences) and it's something I intend to pursue soon.
Summary
This was a strong prediction by the perception-action model and the results were clearly in favour of this approach. This is even more impressive given that the model is not, currently, a model of learning. We cannot actually model these results (or the next ones) yet, although we are working on it. The dynamic pattern hypothesis has no obvious account for these data, nor a reason to even run this experiment.
References
Bogaerts, H., Buekers, M. J., Zaal, F. T., & Swinnen, S. P. (2003). When visuo-motor incongruence aids motor performance: the effect of perceiving motion structures during transformed visual feedback on bimanual coordination. Behavioural Brain Research, 138, 45-57. DOI
Maslovat, D., Hodges, N.J., Krigolson, O. E., & Handy, T. C. (2010) Observational practice benefits are limited to perceptual improvements in the acquisition of a novel coordination skill. Experimental Brain Research, 204, 119-130. DOI
Wilson, A. D., Collins, D. R., & Bingham, G. P. (2005a) Perceptual coupling in rhythmic movement coordination – Stable perception leads to stable action. Experimental Brain Research, 164, 517-528. Download
Wilson, A. D., Collins, D. R., & Bingham, G. P. (2005b) Human movement coordination implicates relative direction as the information for relative phase. Experimental Brain Research, 165, 351-361. Download
Wilson, A.D., Snapp-Childs, W., & Bingham, G. P. (2010). Perceptual learning immediately yields new stable motor coordination. Journal of Experimental Psychology: Human Perception and Performance, 36 (6), 1508-1514 DOI: 10.1037/a0020412 Download
Wimmers, R. H., Beek, P. J., & van Wieringen, P. C. W. (1992). Phase transitions in rhythmic tracking movements: A case of unilateral coupling. Human Movement Science, 11, 217-226. DOI
-
Ecological Mechanisms And Models Of Mechanisms (#mechanismweek 4)
Mechanistic models are great, but so far cognitive science doesn't have any. We have functional models (of, for example, memory or categorisation) and dynamical models (of, for example, neural networks) but none of these can support the kind of explanations mechanistic...
-
Coordination Dynamics And Relative Speed
The Bingham model of coordinated rhythmic movement makes three predictions. First, it predicts that movement stability is a function of perceptual ability, and we confirmed this in two ways (by showing how people can move stably at non-0° with transformed...
-
Visual Feedback For Training Novel Coordinations
The key feature of coordinated rhythmic movements is that not all coordinations are stable. Most other rhythms can be learned, however, which is why we can have jazz drumming. People have been training participants to perform novel coordinations (especially...
-
A Perception/action Model Of Coordination
Other coordination posts are here. The role of perception in the dynamic leading to the HKB phenomena has been made clear by the work so far. But the exact form of this dynamic had yet to be modelled; the HKB model only consists of two cosine functions...
-
Learning A Novel Coordination - The Dynamic Pattern Hypothesis
Other coordination posts are here. Recall this image:The HKB potential function, ~1HzThis basic layout is supposed to describe an intrinsic state of affairs, namely that only 0°and 180°are stable coordinations (+/- 180° are identical except for which...
Neuroscience